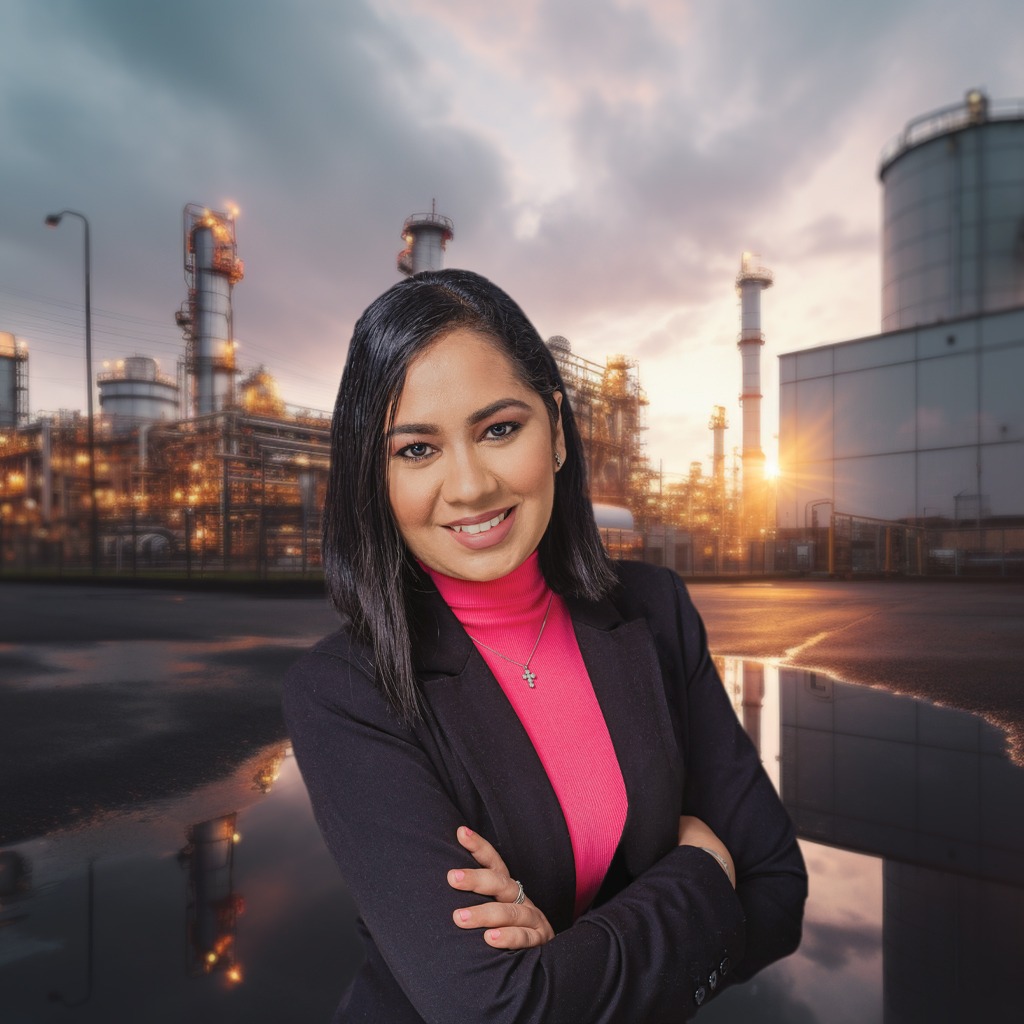
Sanya Mathura, explores the question of why there are so few Registered Female Engineers in Trinidad & Tobago with the Board of Engineering Trinidad & Tobago
Engineer Sanya Mathura, BSc. MSc. MLE, FLCAT I, MAPETT, R.Eng.
For more info on the BOETT check out their website: www.boett.org
As International Women in Engineering Day approaches, we reflect on the number of female registered engineers in Trinidad & Tobago, what may hamper their decision to move forward with this registration and ways to get more women involved in this industry.
With the recent announcement of the expected 11-year lifespan of the oil & gas sector in Trinidad and Tobago, there is a looming question of what the economy will look like in the next two decades. Trinidad and Tobago is not a newcomer to this sector and in fact has over 100 years’ experience in this space. However, with the reserves dwindling and the job security of thousands of people at risk, it is important to plan for the future where all of our citizens can contribute to, and enjoy the benefits of, a thriving economy.
Engineering plays a critical role in the economic development of any country. It underpins the public infrastructure that we utilize daily: roads, water, the Internet and more. It is also the means by which medical and other instrumentation is designed, built and maintained. Engineering can be viewed as one of the foundational pillars of a society. Without a doubt, the integrity of the engineering profession and engineers themselves is therefore critical to our safety and well-being.
Registration with a certifying body, in the case of Trinidad and Tobago, the Board of Engineering, BOETT, validates claims made by engineers regarding their credentials, and that they have satisfied a rigorous assessment of professional commitment as well as competency in accordance with recognized professional standards. Engineers registered with the Board of Engineering of Trinidad & Tobago are accountable to conform to a legislated Code of Ethics in their interactions with the public, employers, and clients; are obliged to protect the public health, safety and welfare; and are called to demonstrate competency, objectivity and confidentiality in all of their professional work.
According to the BOETT, as of 2024, there are 1026 registered engineers, 16% of whom are female. This low percentage is not an anomaly, as there are countless studies which have demonstrated the critical need for gender balance in science, technology, engineering and math (STEM). In this article, we will examine the potential sources of the marked gender imbalance among registered engineers in Trinidad and Tobago, as well as strategies that can be employed to encourage greater levels of registration.

A closer look at the BOETT registration gender split by engineering discipline shows how that 16% or 166 female registered engineers have been distributed.
While the highest number of female registered engineers reside in the Civil Engineering discipline (83), this only accounts for 19% females in that field. On the other hand, Chemical engineering shows a higher percentage of female registered engineers at 37% but this translates to 26 female engineers as shown in Figure 1.
Engineering has been a traditionally male populated industry globally and this trend is also seen in our twin island country. Since the BOETT registration requires 4 years of engineering experience and evidence of further learning equivalent to a Master’s degree, it is critical to examine the trends in propagation from the Bachelor’s to Master’s Degrees.
According to The University of the West Indies[1], the Engineering Faculty has seen a steady instream of undergraduate enrolments over the last five years averaging around 1100 students.
However, not all these students go on to the postgraduate level. In fact, the enrolment values of postgraduates are almost half of the undergraduate students. This trend is evident for the year 2023 where only 31% of the number of undergraduate students who leave the University pursue and attain a postgraduate degree in Engineering as shown in Figure 2.

Upon a deeper dive into the data for the Electrical and Computer Engineering Department, we notice that the number of female undergraduate students generally remains above a 15% threshold for the past 5 years. This is particularly interesting as the percentage of female students continually increases and almost doubles (except for the year in which COVID commenced) as shown in Figure 3.
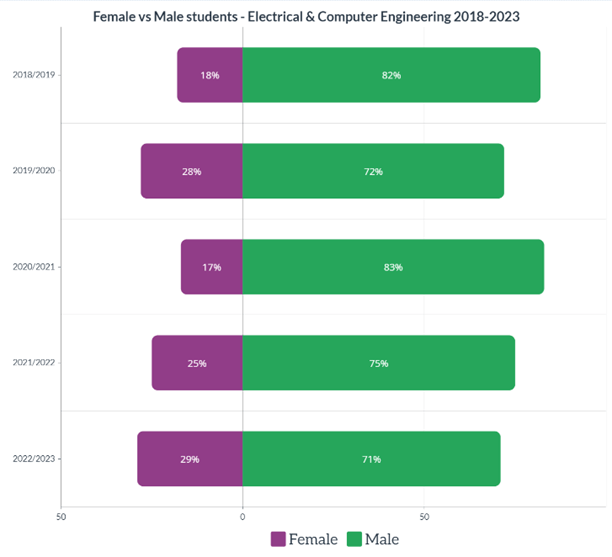
Interestingly enough, when we look at the data for the enrolment of students into Postgraduate Engineering programs it is encouraging to see that there is a higher percentage of women enrolling into these postgraduate programs compared to the undergraduate level as shown in Figure 4 below. Unfortunately, the percentage declines as the postgraduate program continues resulting in a lower overall number of students (both male and female).

There are similar trends in UK and US based Universities. As per the National Center for Science and Engineering Statistics (NCSES)[2] there has been an increase in female students pursuing Engineering degrees in the last 10 years at both the undergraduate and postgraduate levels in the United States of America as shown in Figure 5.


In the United Kingdom according to the Higher Education Student Statistics[3] the percentage of female undergraduate engineering students remained around the same for the last three years (2020-2023), averaging around 17% while the postgraduate female engineering students increased to roughly 27% as shown in Figure 6.
When looking at the actual numbers for the UK, it is quite surprising that the number of undergraduates remains fairly constant with a typical drop off around 300-500 female engineering students to postgraduate studies. However, there is a larger drop with the male students pursuing their postgraduate engineering degrees as per Figure 7 below.

What are some of the challenges faced by women in engineering?
While the numbers for registered female engineers may seem a bit dismal, we need to examine why there’s such a drop off between obtaining an undergraduate degree and becoming a registered engineer. Typically, one qualifies to become a registered engineer only after they have gained an evidential level of engineering competency through work experience in the field. Is this the area where we are losing our female engineers?
Globally, it has been observed that after 5 years within the industry, female engineers usually either leave the discipline entirely or transfer to another non-technical role. There are a number of reasons why this occurs. Based on interviews with many female engineers some of the reasons cited include; lack of basic needs (such as clean bathroom facilities, lactating rooms for new mothers), the presence of microaggressions, lack of safety (especially regarding ill-fitting PPE) and even the basic concept of remaining unheard or unrecognized for their contributions.
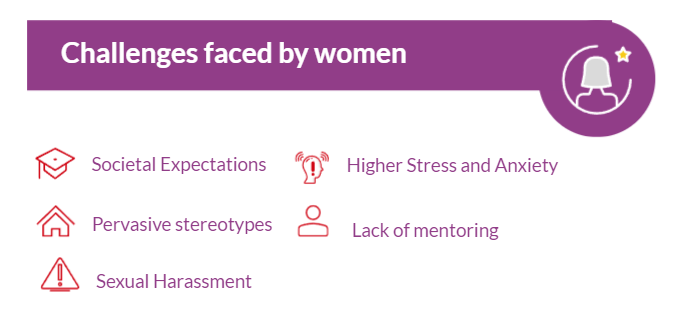
Figure 8 shows an overview of some of the main challenges for women in male populated workplaces. This includes:
•Societal expectations and beliefs about women’s leadership abilities – in these scenarios, women’s voices are almost left unheard, and their contributions are ignored. When trying to lead a team, it may be difficult for them to gain respect of the other team members if the team members do not fully believe in their leadership strategies.
•Pervasive stereotypes, such as that of the “caring mother” or office housekeeper – often, women are assigned these duties in addition to their own job responsibilities which detracts from their time to perform the work assigned to them. Due to these “stereotypes”, they are also not taken seriously in their roles as leaders or when they try to add value to the team as their team members only perceive that they can add value in the stereotypical roles.
•Higher stress and anxiety compared to women working in other fields – women constantly feel the need to always be at their best in these industries. They spend more time working on projects to ensure that they are familiar with every detail as they will be questioned on it and may even have to do the “prove it again” concept where they are asked to prove their findings multiple times before they are believed. In non-male populated environments, women can freely assume leadership roles without the stress or anxiety of whether their work will be questioned.
•Lack of mentoring and career development opportunities – women are often passed over for promotions without the help of sponsors in their organizations. Mentors can also help in creating introductions for women in these fields and aid in their networking to help them in their career development. Mentors play a critical role for women in these fields as they can establish bonds and stronger networks to be considered for other opportunities (within and outside of the organization).
•Sexual harassment – unfortunately, this occurs in the workplace too often especially for women and depending on the circumstances of its occurrence, it can leave the victims fearful of coming to work, which negatively impacts on their performance in the workplace. Additionally, there is also the fear of reporting a senior manager or supervisor for their inappropriate behaviour. The women in these situations may be victimized and even have trouble in reporting the incident as the report would not be taken seriously.
While the list above is not exhaustive, these are just scratching the surface of some of the issues women face in such a male populated discipline.
Coping mechanisms
Quite often this leads to women finding coping mechanisms to deal with some of the challenges listed above. As shown in Figure 9, these include:
•Distancing themselves from colleagues, especially other women – if you realize that this is occurring with one of your female or male colleagues, then check in on them. Find out what you can do to support. Very often, they just need to be supported or not to feel alone in the situation they are facing. Your support could mean the difference between them leaving the industry entirely.
•Accepting masculine cultural norms and acting like “one of the boys,” which exacerbates the problem by contributing to the normalization of this culture – becoming part of the “boys’ club” is not the answer when trying to fit in. Eventually, women lose their authenticity and the unique perspective that they can bring to different situations. It is very important for women in these fields to remain true to themselves and bring their personality to work, that’s what will help us to evolve. This change in the “norm” will help to bring a diverse sense of thinking to create more solutions.
•Leaving the industry - Women sexually harassed at work are 6.5 times as likely to change jobs[4] often to one with lower pay. We are losing our workforce because we’re not standing up for our women who have had this experience. These women feel that they need to leave the industry to be in a safe environment where they are not harassed. We should not have such an unwelcoming environment for women or men.

The aforementioned list is just a few of the coping mechanisms that women have used over time to handle challenges within this industry. If you see one of these mechanisms being used, then take some time to chat with the person.
These coping mechanisms are not just strategies that women use; they can also be used by men. As our brother’s / sister’s keeper, we should look out for each other and continue to support each other.
Finding solutions
Women are entering these male populated fields and changing them for the better. We cannot continue to do things the same way and expect different results. Evolution can only occur if there are significant changes.
Traditionally, jobs were associated with particular genders as these required certain characteristics. For instance, some jobs required physical strength which assumed a male candidate. However, with the advent of technology and tools which can be used by both men and women, many of these jobs now have level playing fields because of these. But society has not caught up with these changes.
As such, women are still faced with challenges in these male populated environments. It is our duty to all work together to create safer environments for women, recognize when there is an issue and come together to solve the issue as a team. This is the only way we can all move forward in these industries.
Currently, we are on the brink of having a major skills gap shortage that the future generation will be responsible for filling. How are we preparing them for these roles? We need to be the change that the future generation sees. If they can “see” more registered female engineers, we can have more female engineers in the future.
Board certification is the only legislated professional credential for engineers practicing in Trinidad and Tobago. For that reason, this credential is most valuable in that it represents, among other things, a commitment to a legislated code of ethics which serves to protect the public interest, elevate the level of professionalism in engineering practice and brings more value and benefits to engineering stakeholders, including the public, clients, employers, and practicing engineers themselves.[5]
The accreditation and verification of experience, knowledge and skills which accompanies registration with the BOETT has the potential to reduce some of the barriers faced by women in these fields.
Generally, with more female engineers, we can expect more inclusive workplaces and an increase in the diversity of thought to create better solutions. Registration strengthens the credibility of practicing engineers especially female engineers.
It is important to the profession and to enable the growth of a community where registration is encouraged, and its value emphasized. Various strategies are required to purposefully empower more women to allow them to drive change in our workplaces and by extension, our lives.
We need to change the conversation towards having a more inclusive workplace for both men and women in engineering. This is the only way we can truly move forward with developing our country and ensuring that our greatest resource (our people) can be a part of that. Let’s get more women and men to become registered engineers in our country.
End notes
[1] (The University of the West Indies | St Augustine Campus, 2023)
[2] (National Center for Science and Engineering Statistics (NCSES), 2023)
[3] (Higher Education Statistics Agency, 2023)
[4] (Blackstone, McLaughin, & Uggen, 2017)
[5] (Lezama, 2024)
References
Blackstone, A., McLaughin, H., & Uggen, C. (2017). The Economic and Career Effects of Sexual Harassment on Working Women. Sage Journals. doi:https://doi.org/10.1177/0891243217704631
Higher Education Statistics Agency. (2023). Higher Education Student Statistics: UK, 2021/2022 - Subjects Studied SB265. Cheltenham, GL50 1HZ: HSEA.
Lezama, V. (2024, June 04). Are you a Board-Registered Engineer? Your career success may depend on it. Trinidad & Tobago.
National Center for Science and Engineering Statistics (NCSES). (2023). Diversity and STEM: Women, Minorities, and Persons with Disabilities 2023, Special Report NSF 23-315. Alexandria, VA: National Science Foundation. Retrieved from https://ncses.nsf.gov/wmpd
The University of the West Indies | St Augustine Campus. (2023). Student Statistical Digest 2018/2019 to 2022/2023. St Augustine: Prepared by the Campus Office of Planning and Institutional Research.
About the author
Sanya Mathura is the Founder of Strategic Reliability Solutions Ltd based in Trinidad & Tobago and operates in the capacity of Managing Director and Senior Consultant. She works with global affiliates in the areas of Reliability and Asset Management to bring these specialty niches to her clients. She holds her BSc in Electrical and Computer Engineering, MSc in Engineering Asset Management and is an ICML certified MLE (Machinery Lubrication Engineer) – the first person in the Caribbean. Sanya was also the first female in the world to achieve the ICML Varnish badges (VIM & VPR) and again the first female globally to attain the Mobius FL CAT I certification (as per their public records). She is also the first engineer to be registered with the Board of Engineering of Trinidad and Tobago in the specialist category of Machinery Lubrication Engineer.
She sits on the Editorial board for Precision Lubrication Magazine and is a digital editor for Society of Tribologists and Lubrication Engineers (STLE)’s TLT Magazine for 2024 and columnist for Equipment Today Magazine. She also sits on the board for the Lubricant Expo North America.
She is the author and co-author of six books; Lubrication Degradation Mechanisms, A Complete Guide, Lubrication Degradation – Getting into the Root Causes, Machinery Lubrication Technician (MLT) I & II Certification Exam Guide and “Preventing Turbomachinery ‘Cholesterol’ – The Story of Varnish.” She has also been assigned the Series Editor of the book series, “Empowering women in STEM” with the first book being launched in Dec 2022, Empowering Women in STEM – Personal Stories and Career Journeys from Around the World and the second in March 2024 called, Empowering Women in STEM – Working Together to Inspire the Future. When not writing or managing the business, you can find her supporting projects to advocate for women in STEM.